The Tesla turbine is a type of turbine designed by inventor Nikola Tesla in the 1890s, characterized by its use of smooth, flat disks instead of conventional blades to harness energy from fluid flow. This innovative design operates on the principles of boundary layer effects and laminar flow, allowing for potentially higher mechanical efficiencies, particularly in smaller applications. The turbine’s unique construction and operational principles differentiate it from traditional turbines, making it a subject of renewed interest in both engineering and renewable energy fields due to its versatility and efficiency.
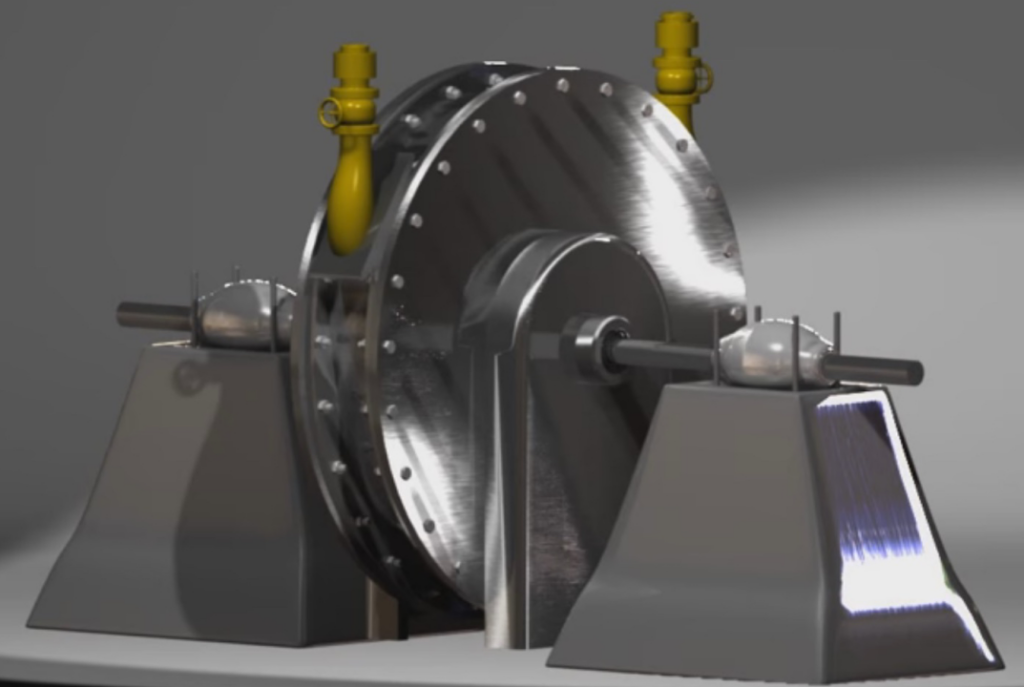
Notably, the Tesla turbine can achieve mechanical efficiencies of up to 97% under optimal conditions, although real-world applications often yield efficiencies of around 35% to 60% depending on various operational parameters. Its ability to function effectively with different types of fluids positions it for diverse applications, including energy generation from renewable sources, industrial processes, and even marine propulsion. However, despite its advantages, the Tesla turbine is not suitable for high-power applications and is sensitive to flow rate variations, limiting its use in certain contexts.
The historical significance of the Tesla turbine lies within the broader context of 19th-century technological advancements and the Second Industrial Revolution, during which Tesla’s innovations contributed to the evolution of electrical systems. While the turbine did not achieve widespread adoption during Tesla’s lifetime, its principles continue to inspire contemporary engineering research, particularly in areas focused on sustainability and efficient energy systems. In recent years, the Tesla turbine has garnered attention as a potential solution for integrating renewable energy technologies, particularly in the face of escalating global energy demands. As interest in alternative energy solutions grows, the Tesla turbine stands as a testament to innovative engineering with promising prospects for the future.
Design and Construction
Overview
The design and construction of the Tesla turbine represent a unique approach to energy conversion, utilizing a series of smooth disks rather than conventional blades. This innovative configuration allows the turbine to harness energy from fluid flow in a more efficient manner than traditional turbine designs. The Tesla turbine operates on the principles of boundary layer effects and laminar flow, which are crucial for its functionality and efficiency.
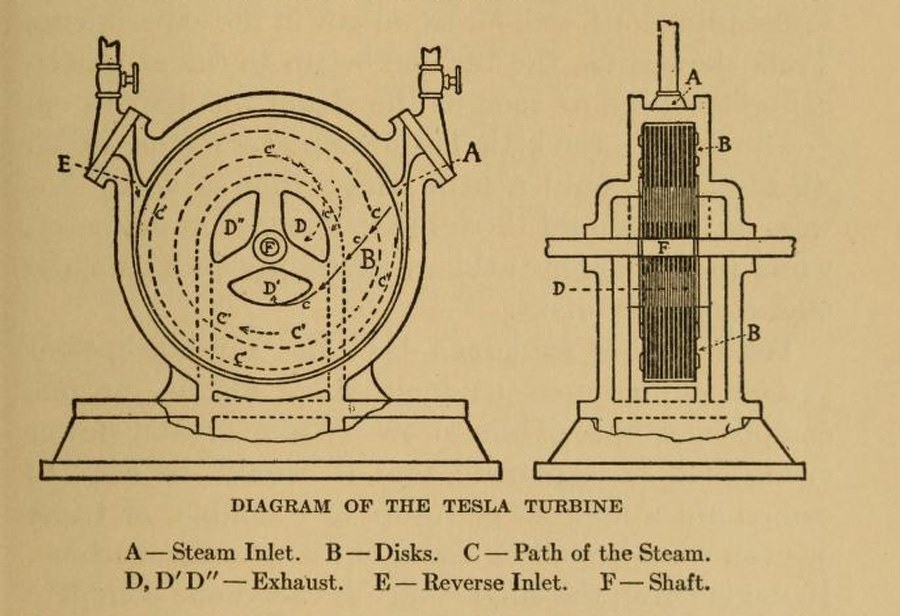
Key Components
Disk Arrangement
The core of the Tesla turbine consists of several flat, parallel disks, which are spaced closely together. The rotor assembly typically includes four rotors, each with a varying number of disks, to optimize performance across different operational parameters. This arrangement minimizes turbulence and promotes smoother flow, enhancing the overall efficiency of the turbine.
Turbine Enclosure
The turbine is housed within an enclosure designed to direct the flow of the working fluid effectively. This enclosure contains nozzles that are adjustable in area, angle, and shape, allowing for fine-tuning of the fluid dynamics as it approaches the disks. This design feature is critical for maximizing the energy extracted from the fluid.
Performance Characteristics
Fluid Dynamics
The operation of the Tesla turbine relies heavily on the principles of fluid dynamics, particularly the boundary layer effect, which describes how fluid velocity changes near a solid surface. In the Tesla turbine, the fluid adheres to the surface of the disks, where it gains energy through viscous shear, a process that is facilitated by the close spacing of the disks.
Efficiency
Experimental designs have demonstrated that Tesla turbines can achieve mechanical efficiencies exceeding 35% across a range of sizes, from 1 mm to 400 mm in diameter. The efficiency is highly sensitive to rotor spacing, input head, and flow variations, making careful design and testing critical for optimal performance.
Construction Techniques
Materials
The construction of the Tesla turbine involves selecting appropriate materials that can withstand the operational stresses while providing the necessary strength-to-weight ratio. Common materials include aluminum and stainless steel, which are favored for their durability and resistance to corrosion.
Manufacturing Methods
The disks and other components can be fabricated using commercially available technologies, including precision machining and 3D printing, which allow for accurate production of the turbine parts. Advanced techniques enable engineers to create complex geometries that enhance the turbine’s performance characteristics.
Operation
The operation of the Tesla turbine is based on the principles of fluid dynamics, specifically utilizing the properties of adhesion and viscosity found in liquids and gases. Unlike traditional turbines that employ blades or vanes, the Tesla turbine relies on smooth discs to generate motion.
Fluid Dynamics Principles
As the working fluid, whether it be air or water, enters the turbine through the inlet nozzle, it is crucial that the fluid maintains a pressure slightly above atmospheric levels. This allows the fluid to flow toward the center of the discs in a laminar manner, minimizing turbulence which can drastically reduce efficiency. The viscosity of the fluid creates a boundary layer effect, where the fluid close to the disc surface adheres and drags along with the movement of the disc, thus transferring energy efficiently.
Boundary Layer Effect
The interaction between the fluid and the discs generates a boundary layer, which is a thin layer of fluid that experiences a velocity gradient. The molecules in this layer move slower than those further away from the surface due to the viscous forces acting against them. As the fluid moves through the discs, it is accelerated and redirected by the boundary layer effect, allowing the turbine to maintain a high efficiency rate of approximately 95% under optimal conditions.
Design Characteristics
The design of the Tesla turbine is critical to its operation. The discs are mounted on a common shaft, and their spacing is crucial for maintaining laminar flow. If the interdisk spacing is too large, the flow may become turbulent, leading to a significant drop in efficiency. The ideal gap size is determined by the peripheral velocity of the discs, which must be optimized to ensure that the fluid experiences minimal resistance and maximal energy transfer.
Energy Transfer Mechanism
As the fluid spirals outwards from the center of the turbine, it gains speed due to the continuous energy transfer facilitated by the boundary layer effect. This acceleration creates a pulling force on the discs, causing them to spin. The efficiency of the turbine is significantly affected by the design choices, particularly the spacing of the discs and the maintenance of laminar flow throughout the operational cycle.
Applications
The Tesla turbine, known for its unique design and efficiency, has several applications across different fields, demonstrating its versatility and innovative engineering.
Energy Generation
One of the primary applications of the Tesla turbine is in energy generation. Its design allows for efficient conversion of heat energy into mechanical energy, which can then be transformed into electrical energy. This application is particularly relevant in contexts where high-temperature sources, such as industrial waste heat or geothermal energy, are available. Moreover, advancements in turbine technology have led to increased turbine sizes while maintaining the fundamental principles established by Tesla, making them a significant source of electric power in various regions.
Renewable Energy Systems
Tesla’s turbine also plays a critical role in renewable energy systems. Its ability to work efficiently with alternative energy sources, such as solar and wind, positions it well within the modern energy landscape. For instance, Tesla’s energy storage solutions, including the Powerwall and Powerpack, are designed to work in conjunction with renewable energy systems by storing excess energy generated from solar panels or wind turbines. This synergistic relationship enhances the overall efficiency and effectiveness of renewable energy utilization, promoting sustainability and reducing reliance on fossil fuels.
Marine Propulsion
In addition to power generation, the Tesla turbine finds application in marine propulsion. Despite competition from internal combustion engines, steam turbines remain an important power source for marine vessels due to their reliability and efficiency in converting thermal energy to mechanical energy. The Tesla turbine’s compact design and operational advantages can further optimize performance in maritime applications.
Industrial Processes
The Tesla turbine can also be utilized in various industrial processes, particularly in systems requiring high-speed rotation. Its unique design minimizes wear and tear, allowing for longer operational lifespans in high-demand settings. Applications in industries such as textiles, where efficient power transmission is crucial, underscore the turbine’s potential to revolutionize operational methodologies.
Advantages and Disadvantages
Advantages of the Tesla Turbine
The Tesla turbine presents several advantages that make it an attractive option for various applications:
- High Efficiency: The Tesla turbine is known for delivering increased efficiency, particularly in smaller applications, where it can reach efficiencies of up to 97%.
- Simple Design: The turbine’s construction is relatively straightforward, which can lead to reduced production costs compared to more complex turbine designs.
- Bidirectional Operation: Unlike many traditional turbines, the Tesla turbine can operate in both clockwise and anticlockwise directions, enhancing its versatility in different applications.
- Compact Size: The design allows for a compact size, making it suitable for various environments where space may be limited.
Disadvantages of the Tesla Turbine
Despite its advantages, the Tesla turbine also has several limitations:
- Limited Power Applications: The turbine is not feasible for high-power applications, which restricts its use in large-scale energy generation.
- Flow Rate Sensitivity: For optimal efficiency, the flow rate must be kept minimal, which may not be practical in all situations, particularly where high throughput is required.
- Dependency on Fluid Dynamics: The efficiency of the Tesla turbine is highly dependent on the inflow and outflow characteristics of the working fluids, which can complicate its application in variable operating conditions.
- Limited Range of Applications: Due to its design and operational limitations, the Tesla turbine has a narrower range of practical applications compared to conventional turbines, often being used primarily in niche areas such as biomedical engineering and as pumps for viscous or shear-sensitive fluids.
Comparisons to Other Turbines
The Tesla turbine operates on distinct principles compared to conventional turbine designs, resulting in different efficiency characteristics and applications. Traditional turbines rely on blades to harness fluid dynamics, while the Tesla turbine utilizes smooth rotor discs that capitalize on the boundary layer effect. This design choice allows for potentially higher efficiencies, particularly in smaller applications, where Tesla turbines can achieve efficiencies of up to 97%. In contrast, modern multiple-stage bladed turbines generally reach efficiencies of 60-70%, with large steam turbines showing efficiencies over 90% in practical applications.
The efficiency of the gas Tesla turbine is estimated to be above 60%, with claims of up to 95% under certain conditions, reflecting a significant performance advantage in specific contexts. This disparity in performance can be attributed to the differences in design and operational principles, as conventional turbines suffer from limitations in temperature and speed due to their reliance on blades and materials that may not withstand high-stress conditions effectively.
Additionally, Tesla turbines demonstrate unique advantages in terms of their operational flexibility. Unlike traditional turbines, which may become blocked or inefficient when processing certain fluids, the Tesla turbine’s design allows it to function effectively as a waste pump in industrial applications, where it can handle debris more efficiently than standard vane-type pumps.
This capability stems from the absence of blades, which can become impeded in conventional designs. Moreover, the efficiency of conventional turbines is heavily influenced by the temperature differential between the intake and exhaust, necessitating robust materials capable of withstanding high temperatures for optimal performance. In Tesla’s time, the lack of advanced materials and aerodynamic theory further constrained the efficiency of traditional turbine designs. Therefore, while both Tesla and conventional turbines have their respective advantages and limitations, the Tesla turbine presents a compelling alternative, particularly in scenarios requiring high efficiency and flexibility in fluid handling.
Historical Developments
The development of the Tesla turbine can be understood within the broader context of the significant technological advancements of the 19th century. This period marked a transformative era in engineering and industrialization, characterized by innovations such as the electric light bulb, steam engines, and advanced power distribution systems. These advancements played a crucial role in shaping modern society, impacting transportation, manufacturing, and energy production. Nikola Tesla, who was active during this transformative period, made substantial contributions to the understanding and application of alternating current (AC) electricity, which revolutionized electrical systems. His invention of the Tesla turbine in the 1890s exemplified his innovative spirit.
Unlike traditional turbines that relied on blades to generate movement, Tesla’s design utilized smooth discs and the principles of boundary layer physics to achieve efficient energy conversion This approach not only demonstrated a new method of harnessing mechanical power but also reflected the broader trends of experimentation and ingenuity of the time. Tesla’s turbine design emerged amidst the backdrop of the Second Industrial Revolution, which saw the widespread use of electricity, steel, and petroleum, fundamentally altering industries such as transportation and manufacturing. The turbine itself was intended to be versatile, capable of utilizing various energy sources, including steam and gas, which aligned with the growing demand for more efficient and adaptable technologies during the late 19th century.
Although Tesla’s turbine did not achieve the widespread adoption that some of his other inventions did, it has garnered renewed interest in modern engineering and renewable energy applications, reinforcing the enduring legacy of 19th-century innovations and Tesla’s pioneering work. The principles behind the Tesla turbine continue to inspire contemporary research into efficient energy systems and alternative power generation methods, illustrating the lasting impact of 19th-century engineering on today’s technological landscape.
Can you explain how the design of the Tesla turbine affects laminar and turbulent flow, and why these flow types are significant for its efficiency?
The design of the Tesla turbine significantly impacts both laminar and turbulent flow, which are crucial for its efficiency. The Tesla turbine operates using smooth discs and relies on the boundary-layer effect rather than the traditional blade method used by conventional turbines. This design choice leads to the presence of different flow characteristics that directly affect the turbine’s performance. The boundary-layer effect in the Tesla turbine is where the fluid moves tangentially across the smooth surfaces of the discs. When a fluid is introduced into the turbine through nozzles, it adheres to the surface of the discs due to viscosity.
This results in a thin layer of fluid, known as the boundary layer, adhering to the disc surfaces. The boundary-layer effect is typically associated with laminar flow, where the fluid moves smoothly and in parallel layers without much mixing between them. This type of flow is essential for the initial stages of fluid interaction with the discs, allowing for efficient momentum transfer with minimal energy loss. As the fluid progresses through the turbine and encounters subsequent discs, it experiences a gradual increase in velocity and centrifugal forces. This can potentially transition the flow from laminar to turbulent.
Turbulent flow is characterized by chaotic and irregular fluid motion, which can increase energy dissipation and reduce efficiency if not managed properly. However, the Tesla turbine’s design aims to maintain a balance between these two flow types to optimize performance. The smooth, close-packed arrangement of the discs helps in sustaining the boundary-layer flow for a longer duration, thereby enhancing efficiency. The significance of these flow types lies in their impact on energy transfer and turbine efficiency. Laminar flow is generally more desirable in the Tesla turbine because it allows for a more predictable and less energy-dissipative interaction between the fluid and the discs. This leads to a more efficient conversion of fluid kinetic energy into rotational energy.
On the other hand, excessive turbulent flow can lead to increased frictional losses and reduced efficiency. Thus, the Tesla turbine’s design strategically manages the transition between laminar and turbulent flow to maximize performance and minimize energy losses. In summary, the Tesla turbine’s unique design, which leverages the boundary-layer effect, significantly affects the behavior of laminar and turbulent flows within the turbine. Maintaining laminar flow as long as possible is crucial for efficient operation, while the transition to turbulent flow must be carefully controlled to minimize energy dissipation and optimize the turbine’s overall efficiency.
What are the most common fluids used in Tesla turbines, and how do their properties influence the turbine’s efficiency?
The most common fluids used in Tesla turbines are steam and air, though various other fluids can also be utilized depending on the application. The properties of these fluids significantly influence the turbine’s efficiency due to the unique operating principle of the Tesla turbine, which relies on the boundary-layer effect rather than impinging fluid on blades as in conventional turbines.
1. Steam: Steam is a prevalent fluid used in Tesla turbines due to its high energy content and ease of generation. When steam is used, it enters the turbine at high velocity through nozzles, impacting the smooth discs arranged similarly to a stack of CDs on an axle. The momentum exchange between the steam and the discs generates rotational movement. The efficiency of steam in Tesla turbines can be high, particularly at optimal temperatures and pressures. According to source, in a specific application, a Tesla steam turbine operating at 9,000 revolutions per minute and with 125 pounds at the throttle was able to develop 200 horsepower, demonstrating its potential for high efficiency under suitable conditions.
2. Air: Air can also be used in Tesla turbines, especially in applications where steam is impractical. The efficiency of air as a working fluid is generally lower compared to steam due to its lower energy density. However, air turbines can still be effective in scenarios where simplicity and lower operational temperatures are desired.
3. Other Fluids: While steam and air are common, other fluids can be used depending on the specific requirements and environmental conditions. For instance, geothermal power applications might use hot gases or vapors from geothermal sources. Tesla envisioned his turbine being used in geothermal power, as highlighted in source. The efficiency of the Tesla turbine is influenced by the fluid’s properties such as viscosity, temperature, and pressure. The boundary-layer effect, which is central to the turbine’s operation, is more effective with fluids that can maintain a laminar flow along the disc surfaces.
Higher viscosity fluids can enhance the interaction between the fluid and the discs, thereby improving momentum transfer and rotational efficiency. Overall, while the Tesla turbine can operate with various fluids, the choice of fluid and its properties are crucial in determining the overall efficiency and effectiveness of the turbine in different applications.
What notable experiments or applications of the Tesla Turbine were conducted during Tesla’s time or afterward?
During Nikola Tesla’s lifetime, he conducted several notable experiments and envisioned various applications for his Tesla turbine, although widespread adoption of his invention did not materialize during his time. Tesla first built a working model of the turbine in 1906. This early prototype was compact, weighing less than 10 pounds and measuring only six inches in length, yet it was capable of generating 30 horsepower.
In 1913, Tesla patented the Tesla turbine, which he regarded as “the greatest of all his inventions”. The innovative design of the turbine, which utilized smooth, parallel discs instead of blades, was based on the boundary-layer effect, where the fluid’s momentum is transferred to the discs, generating rotational movement. Tesla envisioned several practical applications for his turbine. He believed it could be used in vehicles and other high-practicality scenarios, and even suggested its potential for geothermal power generation.
Additionally, Tesla aimed to develop a lighter engine for a “flying machine” using the turbine’s design. Despite its promising design and Tesla’s high regard for the invention, the Tesla turbine did not gain widespread use due to the entrenched dominance of piston engines in the automotive industry and the substantial investments already made in Parsons and Curtis turbine designs by major electrical production companies like GE and Westinghouse.
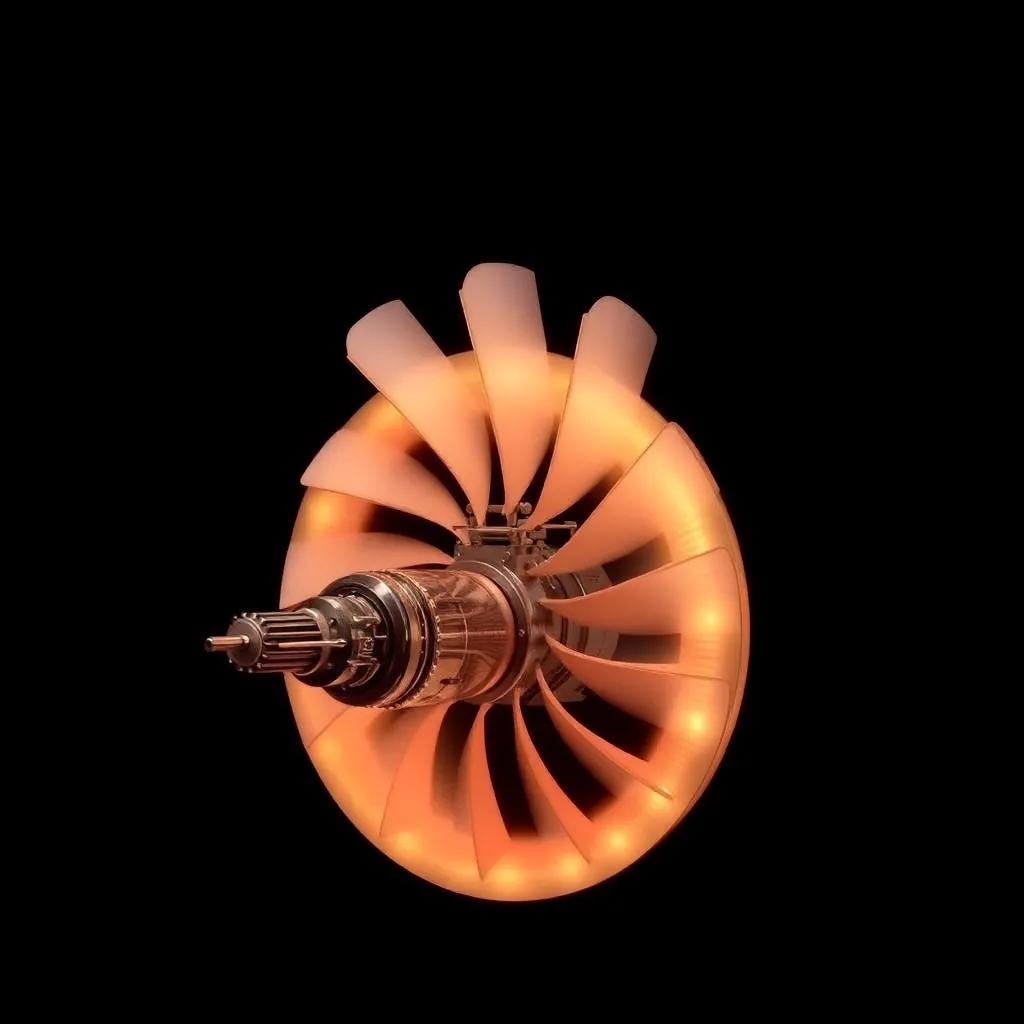
In later years, interest in the Tesla turbine has been revived, and it has been researched for various modern applications. Bioengineering researchers have referred to it as a multiple-disk centrifugal pump, exploring its potential in new fields. Overall, while Tesla’s own experiments and the turbine’s initial applications were limited by the technological and industrial context of his time, the concept has continued to capture the interest of researchers and engineers, hinting at its potential for future innovations.