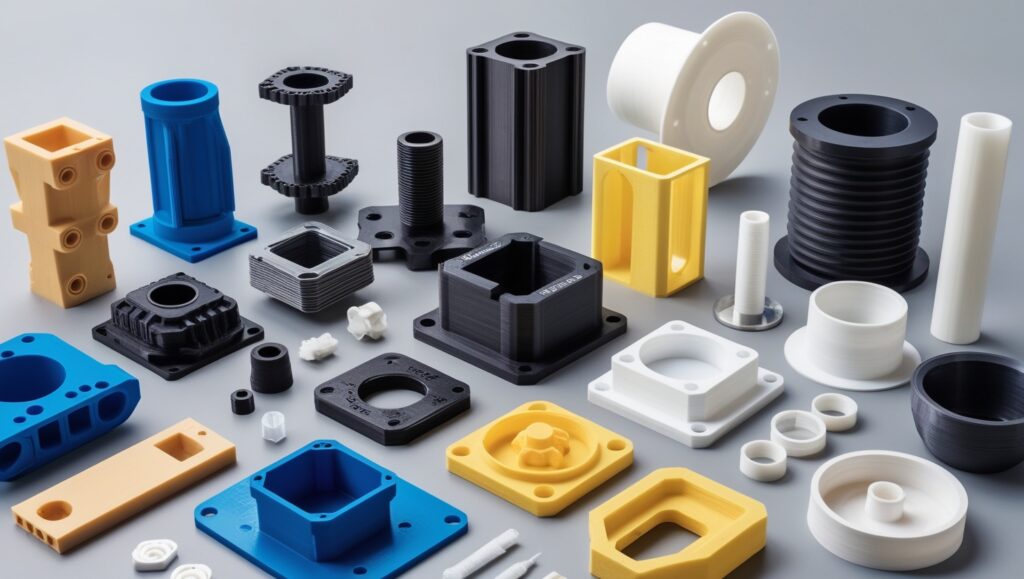
How To Strengthen 3D Printed Parts
Strengthen 3D printed parts is a vital consideration in additive manufacturing, as these components are often perceived to be less robust compared to traditionally manufactured items. Various methods, including material selection, design adjustments, and post-processing techniques, can significantly enhance the strength and durability of 3D printed parts. This topic is notable due to the growing adoption of 3D printing across multiple industries, such as aerospace, automotive, and healthcare, where performance and reliability are paramount. One of the primary strategies for improving strength is the careful selection of materials.
High-quality filaments like ABS, Nylon, and PETG provide better mechanical properties than standard materials like PLA, making them more suitable for load-bearing applications. Additionally, factors such as part orientation during the printing process and wall thickness play crucial roles in determining the overall structural integrity of the final product. For example, optimizing part orientation can minimize weak interfaces between layers, while increasing wall thickness can provide extra support and durability. Post-processing treatments, such as epoxy coating, can further enhance the strength of 3D printed parts by creating composite materials that improve overall finish and resistance to mechanical stress.
Understanding the effects of infill density, layer height, and printing parameters is also essential for maximizing the performance of printed components, as these settings directly impact layer adhesion and material distribution within the part. Controversies surrounding the strength of 3D printed parts often revolve around varying quality and consistency due to differences in manufacturing practices and materials. Standardized testing and adherence to performance metrics are crucial for comparing material properties and ensuring that 3D printed components meet industry-specific requirements. As the technology continues to evolve, the focus on enhancing the durability of 3D printed parts will be critical for expanding their applications and reliability in demanding environments.
Methods for Strengthen 3D Printed Parts
3D printed parts are often perceived as weak and unsuitable for load-bearing applications; however, various methods can be employed to enhance their strength and durability. These techniques involve adjustments during the design and printing phases, as well as post-processing treatments.
Material Selection to Strengthen 3D Printed Parts
Choosing a high-quality filament is essential for creating strong 3D printed parts. Materials such as ABS or Nylon offer greater strength compared to standard PLA. The choice of material can depend on the specific application and the capabilities of the 3D printer being used, as some materials may require specialized equipment.
Post-Processing Techniques for Strengthen 3D Printed Parts
Epoxy Coating
Applying an epoxy coating to the printed part can significantly increase its strength. This technique involves layering fiberglass and saturating it with epoxy resin to create a composite material. This method not only adds strength but also improves the overall finish and durability of the part. By implementing these methods, users can significantly enhance the performance and longevity of 3D printed parts, making them suitable for a wider range of applications beyond prototyping and hobbyist projects.
Design Considerations in Strengthen 3D Printed Parts
Part Orientation
Part orientation is a critical factor in the strength of 3D printed components. Since the layers are deposited in the Z-direction, the interfaces between layers can be weak points. Properly orienting the model during the slicing process can help mitigate this issue by aligning the weaker sections along the X-Y plane, thereby reducing the likelihood of failure under mechanical stress.
Wall Thickness and Shells
Increasing the wall thickness or the number of shells (perimeters) around the part can significantly improve its structural integrity. The shell thickness setting determines the width of the outer walls, which directly influences the part’s strength. A thicker shell provides additional support, while also improving the overall durability of the print.
Printing Parameters in Strengthen 3D Printed Parts
Infill Density and Pattern
The choice of infill density and pattern can greatly affect the strength of the printed part. Higher infill percentages provide more material, which can enhance strength; however, values above 75% may not yield significant improvements in some cases. A 50% infill can sometimes provide similar strength to a 100% infill, making it a more efficient choice in terms of material and print time.
Layer Height
Reducing the layer height during printing can improve the detail and strength of the final product. Finer layers create better layer adhesion, which can enhance the overall durability of the part. This approach also allows for greater control over the final appearance of the print.
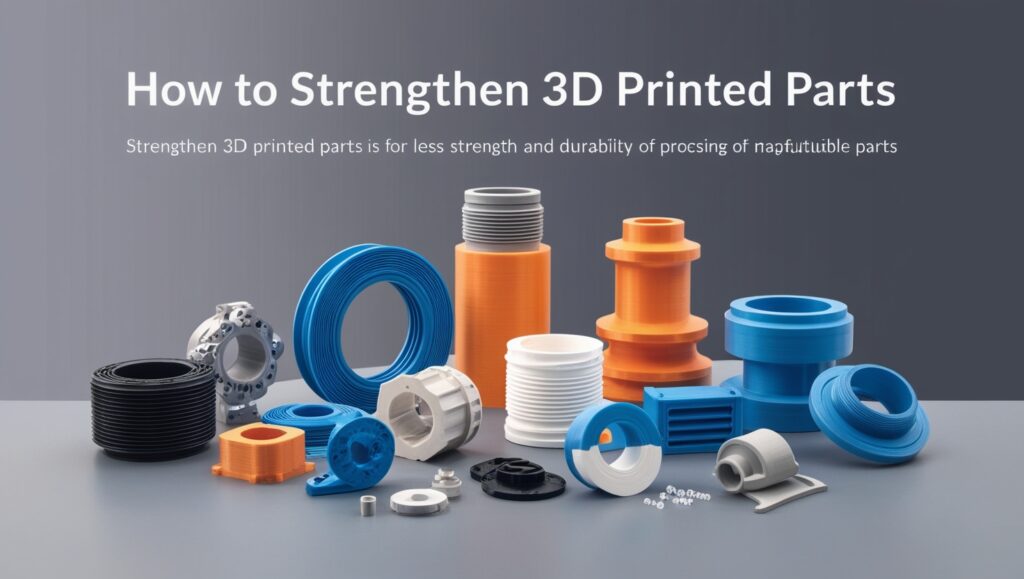
Strength Testing
Impact Strength
Impact strength refers to the amount of energy, typically measured in kilojoules per square meter (kJ/m²), that a material can absorb without fracturing. Brittle materials exhibit low impact strength, making them prone to breaking under sudden forces. In contrast, various filaments such as ABS, PETG, polycarbonate (PC), and flexible materials like thermoplastic polyurethane (TPU) are noted for their high impact resistance. Conversely, polylactic acid (PLA) has been identified as a weaker material in this regard, often shattering upon impact.
Flexural Strength
Flexural strength, or bending strength, assesses a material’s resistance to bending and deformation under load. This property is particularly relevant for applications requiring materials to endure flexing forces, such as structural components and brackets. Flexural strength is quantified in megapascals (MPa) through standardized testing, specifically the three-point bend test, where a specimen is subjected to load until it fractures. Materials like PC, nylon, and fiberglass-reinforced filaments tend to exhibit high flexural strength, thereby reducing the likelihood of deformation or failure under bending stresses.
Tensile Strength
Tensile strength measures the maximum stress that a material can withstand while being stretched before breaking. This property is critical in determining how materials perform under tensile loads. Tensile strength is assessed using a tensile testing machine that stretches a sample until it fails, recording the maximum force applied. Common ranges for tensile strength vary widely depending on the material, with high-strength materials being crucial for load-bearing applications. Notably, research indicates that the tensile strength of 3D-printed parts can be influenced by layer thickness and other printing parameters, which highlights the importance of controlled testing conditions.
Tear Strength
Tear strength evaluates a material’s resistance to tearing, an essential property in applications requiring durability against dynamic stresses. The measurement is often used in conjunction with other tests to comprehensively evaluate material performance, particularly in flexible or elastomeric materials.
Heat Resistance
Heat resistance, often measured by heat deflection temperature (HDT), assesses at what temperature a material begins to deform under load. Testing typically involves gradually heating a sample while applying a specific load to monitor the temperature at which it bends significantly. This property is particularly important for materials exposed to high temperatures in practical applications, as thermal stability can significantly influence their overall performance and longevity.
Importance of Testing Standards
The diversity of testing methods used across different materials and manufacturers can lead to discrepancies in reported strength values, complicating the comparison of materials. To mitigate these challenges, standardized testing methods are essential for providing reliable and consistent evaluations of material properties, enabling better-informed material choices during the design and production of 3D-printed parts.
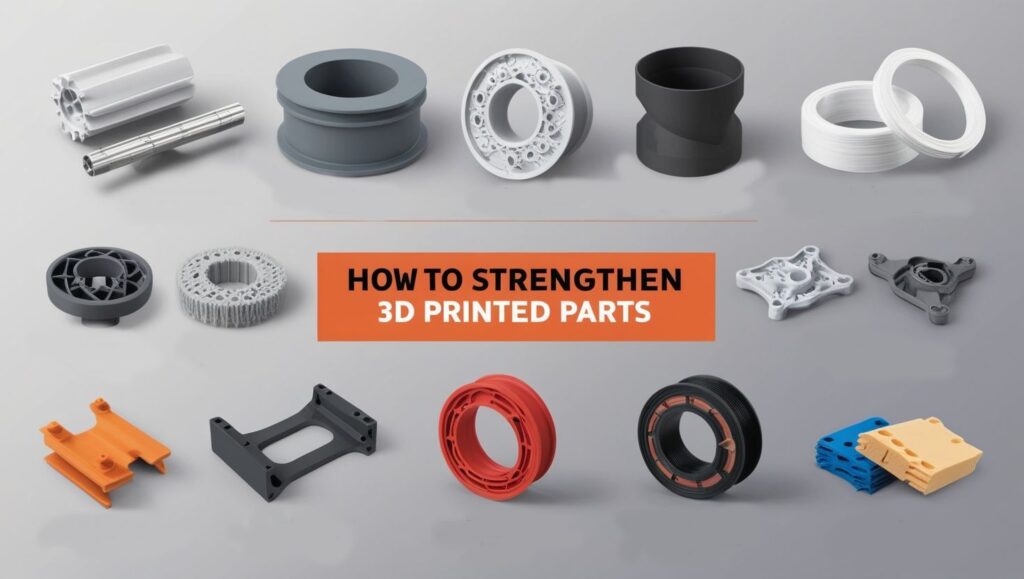
Applications
Additive manufacturing (AM) has a broad range of applications across various industries, leveraging its unique capabilities to create functional and customized components.
Medical Applications
In the medical sector, AM is instrumental in producing patient-specific implants, prosthetics, and biocompatible structures, thus revolutionizing treatment options and healthcare outcomes. The ability to tailor these components to individual patients enhances both the efficacy and comfort of medical treatments.
Automotive Industry
Automotive companies utilize AM for rapid prototyping and the creation of customized parts, significantly streamlining supply chains. This technology allows for quicker design iterations, enabling manufacturers to adapt to changes efficiently and meet specific performance criteria without extensive tooling alterations.
Architectural and Design Innovations
Architects and designers explore AM’s potential to fabricate intricate structures and models, pushing the boundaries of 3D design and fabrication. The adaptability of AM allows for the realization of complex geometries that were previously challenging to achieve using traditional methods.
Food Industry
The food sector has also begun to experiment with AM, developing novel culinary experiences and personalized nutrition solutions. This application showcases the versatility of AM technology beyond conventional manufacturing, opening new avenues for creativity and customization in gastronomy.
Environmental Sustainability
One of the most promising aspects of AM lies in its potential to reduce material waste compared to traditional manufacturing processes. By constructing objects layer by layer and using only the necessary materials, AM promotes sustainability and minimizes excess material generation, aligning with contemporary demands for environmentally friendly manufacturing practices.
Post-Processing and Material Innovations
As industries increasingly adopt AM, there is a growing emphasis on optimizing post-processing techniques to enhance the performance and quality of AM-produced components. Innovations in materials, such as tailored abrasive media and eco-friendly support materials, are being developed to address specific post-processing challenges while reducing environmental impacts. This focus on materials innovation ensures that AM components not only meet but exceed the stringent quality benchmarks required across diverse sectors.
Real-World Case Studies
Aerospace Innovations
The aerospace industry demands materials that can withstand extreme conditions, and additive manufacturing is rising to the challenge. For example, the integration of advanced materials such as Titanium and Carbon Fiber Reinforced Polymer enables the creation of lightweight yet robust components essential for aircraft performance. These materials are critical for constructing intricate interiors and crucial components of jet engines, ensuring that they meet the stringent demands of flight with both durability and precision.
Automotive Industry Applications
In the automotive sector, companies are increasingly leveraging additive manufacturing (AM) to enhance the performance and durability of parts. For instance, Ford replaced a traditional quarter glass alignment fixture with a new Fused Deposition Modeling (FDM) 3D-printed tool. This new tool not only offered a weight reduction of 15% but also resulted in 70% cost savings compared to conventional manufacturing methods. Furthermore, Volkswagen utilized 3D printing to produce complex multi-material PolyJet prototypes, achieving up to 99% precision in mimicking final production parts, which led to a reduction in the product development cycle by two months while allowing for complete design freedom.
Medical Advancements
In the medical field, AM has transformed the production of patient-specific implants and prosthetics, capitalizing on its ability to create biocompatible structures that significantly improve healthcare outcomes. Researchers, including those from Washington State University, have developed lifelike 3D-printed models of human organs. These models, which require careful optimization of geometric precision, weight, and porosity, are invaluable for surgical training and evaluating implant devices. The use of an AI technique called Bayesian Optimization has allowed for enhanced printing settings, resulting in models that closely replicate the mechanical properties of actual organs.
Performance Metrics
Overview of Strength Characteristics
Strength in 3D printed parts is a crucial factor that encompasses various mechanical properties essential for performance and durability. Among these properties, tensile strength, which measures a material’s resistance to breaking under tension, plays a significant role, especially for load-bearing applications. Flexural strength, on the other hand, indicates a filament’s ability to withstand bending without permanent deformation, making it vital for designs subjected to dynamic stressors.
Impact Strength
Impact strength is defined as the amount of energy a material can absorb without breaking, typically measured in kilojoules per square meter (kJ/m²). Materials can exhibit high impact strength while having low tensile strength, leading to varying performance characteristics under different stress conditions. For example, materials like ABS, PETG, and PC are noted for their superior impact resistance, whereas PLA is generally more brittle, making it less suitable for applications where impact resistance is critical.
Retraction Settings and Calibration
The performance of 3D prints can also be influenced by specific settings, such as retraction speed and length. Calibrating these settings properly ensures that the filament retracts at optimal rates during printing, which can prevent issues such as stringing and blobs on the print surface. Techniques like printing a retraction tower help users identify the best retraction settings for their specific filament and printer setup.
Mechanical Components and Their Influence
The mechanical components of a 3D printer, including stepper motors and mechanical assemblies like belts and pulleys, directly impact the accuracy and precision of layer deposition. Lower resolution stepper motors may lead to inconsistent layer heights, which can ultimately affect the overall strength of the printed part. It may be advisable to adjust layer heights to improve consistency and mechanical durability when dealing with such limitations.
Reinforcement Techniques
Effective reinforcement strategies can significantly enhance the strength of 3D printed parts. Techniques include strategically reinforcing areas based on loading conditions and balancing reinforcement across multiple axes to prevent warping. For instance, implementing isotropic fiber reinforcement can strengthen parts against shear and tensile forces, while also allowing for better distribution of loads across the structure.
Balancing Strength, Resolution, and Print Time
When optimizing for strength, it’s essential to find a balance between mechanical durability, surface finish, and print time. Although lower layer heights typically increase strength, they also result in longer print times, which may not always justify the marginal gains in strength. Therefore, a strategic approach is necessary to achieve the desired performance without excessive delays. By understanding and effectively applying these performance metrics and techniques, users can significantly enhance the quality and durability of their 3D printed parts.Show as PDF
Somebody essentially help to make significantly articles Id state This is the first time I frequented your web page and up to now I surprised with the research you made to make this actual post incredible Fantastic job