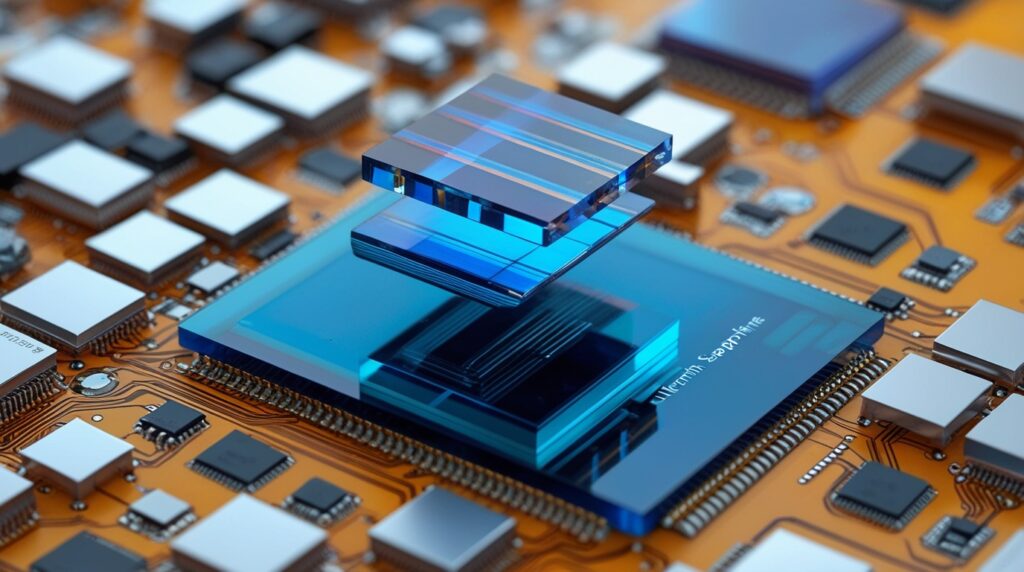
Introduction
Ultrathin sapphire synthesized for the advancement of next-generation transistors, represents a cutting-edge material in the evolution of miniaturized semiconductor chips. This innovative approach addresses the limitations of traditional silicon-based transistors as device dimensions shrink, aiming to enhance electrical performance and efficiency in electronic applications. With remarkable properties such as high dielectric strength, excellent thermal stability, and superior mechanical durability, ultrathin sapphire is positioned to play a crucial role in the development of flexible and high-density electronic devices, including those used in the Internet of Things (IoT) and wearable technology.
The synthesis of ultrathin sapphire employs various advanced techniques, including Chemical Vapor Deposition (CVD) and Metal-Organic Chemical Vapor Deposition (MOCVD), enabling the creation of films that can be integrated with existing silicon infrastructure. These methods allow for the production of ultrathin materials with enhanced electrical characteristics, which are essential for overcoming the challenges posed by Moore’s Law—an industry trend that anticipates the doubling of transistors on microchips approximately every two years. Moreover, innovative fabrication processes have led to defect-free, single-crystalline 2D materials that exhibit superior conductivity compared to conventional bulk materials.
Despite its potential, the development of ultrathin sapphire faces significant challenges, including complex manufacturing processes and defect management, which can compromise device performance. Additionally, the integration of these materials with flexible substrates remains a critical hurdle, as traditional high-temperature techniques are often incompatible with such materials, necessitating new approaches to ensure effective performance and scalability. As researchers continue to address these obstacles, the prospects for ultrathin sapphire in future electronic applications remain promising, potentially revolutionizing the landscape of modern electronics.
Properties of Ultrathin Sapphire
Ultrathin sapphire exhibits a variety of properties that make it suitable for advanced applications in electronics and photonics, particularly in the context of 2D materials and miniaturized chips.
Electrical Properties
Sapphire’s electrical properties are noteworthy, featuring a bulk resistivity of 10¹⁶ ohm-cm at room temperature, which decreases to 10¹¹ ohm-cm at elevated temperatures (500°C) . The dielectric strength is under 48 kV/mm, making it an excellent insulator. The dielectric constant varies between 9.4 and 11.6 depending on orientation, while the loss tangent remains low, at 3.0 – 8.6×10⁻⁵ over a frequency range of 10 Hz to 3×10⁹ Hz.
Optical Properties
Ultrathin sapphire is known for its excellent optical characteristics, boasting a transmittance of over 85% in the 0.3-4.0 µm range for thicknesses around 0.1 mm. The refractive indices are 1.768 for the ordinary ray and 1.760 for the extraordinary ray, yielding a birefringence of 0.008. Its high emissivity at elevated temperatures (up to 70% at 500°C for specific wavelengths) further demonstrates its potential for photonic applications.
Mechanical Properties
Ultrathin sapphire is characterized by remarkable mechanical strength. It has a hardness of 9 on the Mohs scale, translating to approximately 2000 kg/mm² when measured using the Knoop hardness test. Its high flexural strength of 900 MPa and compressive strength of 2.0 GPa further enhance its utility in high-stress applications. The material also exhibits a low coefficient of friction (0.14 on steel), making it advantageous for applications requiring reduced wear.
Thermal Properties
Thermal stability is another key feature, with a melting point around 2053°C (3727°F) and optimal performance up to approximately 1800°C (3272°F). The thermal conductivity is recorded at 40 W/M°K at room temperature, allowing for efficient heat dissipation in electronic applications. Additionally, the material’s low thermal expansion coefficient (4.5×10⁻⁶/K at 25°C) minimizes dimensional changes with temperature fluctuations.
Chemical and Physical Properties
From a chemical standpoint, ultrathin sapphire is non-porous and resistant to various solvents and acids at room temperature. It remains largely unaffected by weathering and hydration, making it suitable for harsh environments. Its density is measured at 3.98 g/cm³, which contributes to its durability. These properties underscore the versatility and effectiveness of ultrathin sapphire in next-generation transistors and other critical applications in miniaturized chips.
Synthesis Techniques
Chemical Vapor Deposition (CVD)
The layering technique known as chemical vapor deposition (CVD) is commonly used for growing films of materials such as molybdenum disulfide (MoS2). In this process, a film is built one layer of atoms at a time, typically requiring high temperatures, often exceeding 850 °C (over 1500 °F). However, this presents challenges when working with flexible substrates like polyimide, which can degrade at significantly lower temperatures. To address this, researchers have developed methods to pattern and form the necessary components on rigid silicon substrates, allowing for the safe transfer to flexible materials without damage.
Dry Synthesis Approach
Recent advancements in the synthesis of ultrathin films have introduced a novel dry synthesis technique that leverages physical vapor deposition and low-temperature plasma treatment. This method circumvents traditional challenges associated with solution processing and the use of harsh chemicals, significantly reducing material and processing costs by eliminating the need for sacrificial metal elements. The versatility of this approach allows for the integration of various metals and compositions, enabling the creation of ultrathin films with thicknesses under 20 nm that can be effectively handled and transferred without collapsing, thereby maintaining their conductivity after integration with existing sensor technologies and device architectures.
Metal-Organic Chemical Vapor Deposition (MOCVD)
Another method employed in the synthesis of thin films involves metal-organic chemical vapor deposition (MOCVD). This technique uses metal-organic precursors that vaporize and decompose at elevated temperatures, typically above 550 °C. However, silicon circuits begin to degrade at temperatures surpassing 400 °C, creating a need for innovative approaches. Researchers have designed specialized furnaces that allow for the successful application of MOCVD while minimizing temperature-related damage to underlying silicon circuits. This method facilitates the growth of uniform thin films, further pushing the boundaries of integrating 2D materials into semiconductor applications.
Applications in Transistor Development
The exploration of ultrathin materials, particularly 2D materials such as transition-metal dichalcogenides (TMDs), has garnered significant interest in the development of next-generation transistors. Traditional silicon-based transistors face limitations as device dimensions shrink below certain thresholds, prompting researchers to investigate alternative materials that can maintain electrical performance at the nanoscale.
2D Materials and Their Advantages
2D materials, which consist of single or few layers of atoms, exhibit superior electronic properties compared to conventional bulk materials. Unlike polycrystalline materials, where grain boundaries impede electron flow, 2D materials can potentially be engineered to have single-crystalline characteristics, thereby enhancing conductivity and reducing energy losses. This property is crucial for achieving smaller transistors that align with the ongoing demands of Moore’s Law, which posits that the number of transistors on microchips doubles approximately every two years.
Innovations in Fabrication Techniques
Recent advancements have led to the development of methods that allow for the growth of defect-free, single-crystalline 2D materials on silicon wafers. This breakthrough was achieved through a technique known as “non-epitaxial, single-crystalline growth,” enabling the fabrication of transistors that are smaller than a few nanometers. The implications of this are profound, as it resolves a long-standing issue in the semiconductor industry: the challenge of integrating 2D materials with existing silicon infrastructure.
Furthermore, researchers at Stanford University have introduced a novel approach to producing flexible, atomically thin transistors. By employing a multi-step fabrication process starting on rigid substrates, they achieved transistors less than 100 nanometers in length, significantly enhancing the potential for flexible electronics. This technique not only demonstrates the feasibility of high-density, low-power devices but also aligns with emerging applications in wearable technology and IoT devices.
Future Prospects
The development of ultrathin sapphire synthesized for next-generation transistors offers promising avenues for advancements in miniaturized chips. Researchers are optimistic about utilizing two-dimensional (2D) materials, such as transition metal dichalcogenides, which have demonstrated superior electrical properties compared to traditional silicon when scaled down to the nanoscale. This shift is crucial as the limits of silicon technology approach a plateau due to inherent electrical property degradation in smaller devices.
The innovative manufacturing techniques, including the recent breakthroughs in producing flexible, atomically thin transistors, further enhance the viability of these 2D materials in practical applications. These advancements enable the creation of circuits that are not only ultrathin—around 5 microns thick—but also capable of handling high electrical currents at low voltage, which is essential for energy-efficient operation in a variety of devices, including wearable technology.
Furthermore, the potential for integrating radio circuitry into these flexible devices opens up new possibilities for wireless communication, particularly for applications in the emerging Internet of Things (IoT) ecosystem. As these technologies mature, the combination of flexibility, density, and high performance is expected to propel the next generation of electronic devices, making them more versatile and widely applicable in fields ranging from healthcare to consumer electronics.
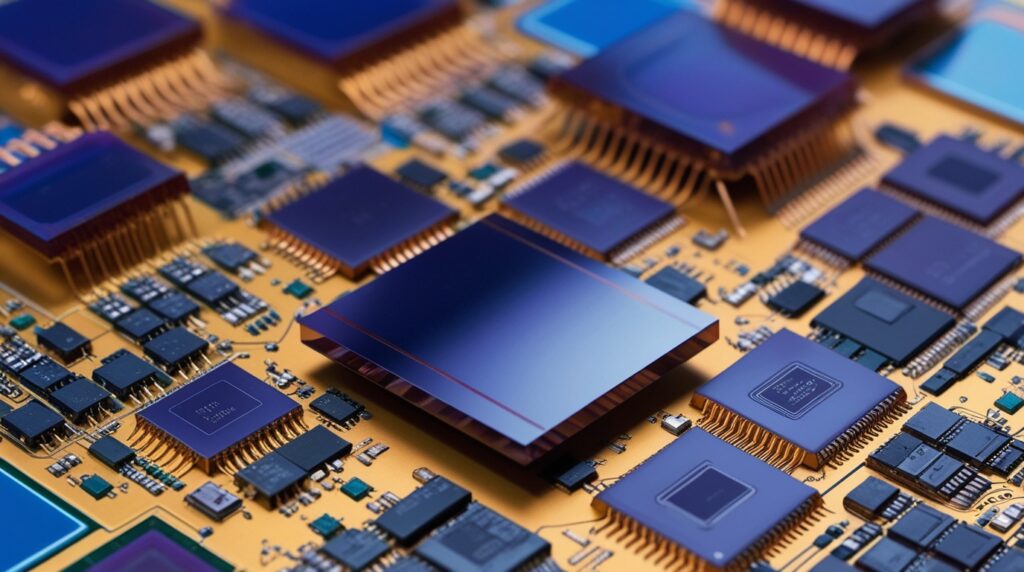
Challenges and Limitations
The development of ultrathin sapphire for next-generation transistors faces several significant challenges that can impact both performance and manufacturability.
Process Complexity
The Eltran (circledR) process, utilized for epitaxial growth of silicon layers on porous structures, inherently reflects surface roughness, necessitating extensive planarization to achieve the smoothness of bulk wafers. However, such surface treatment can reduce film thickness and degrade in-plane uniformity, thereby complicating the manufacturing process. Additionally, the occurrence of stacking faults during the epitaxy can further compromise the quality of the device layer, requiring meticulous fine-tuning of each step involved in the process.
Defect Management
In contrast to the Eltran method, SIMOX technology also faces issues related to defect density and management. High-energy ion implantation essential for this process introduces lattice defects that can adversely affect the performance characteristics of the final device. The presence of oxide precipitates and silicon pipes within the buried oxide (BOX) layer poses additional challenges during fabrication, necessitating advanced refinement techniques to mitigate these defects effectively.
Wafer Bonding Quality
For processes such as Smart Cut™, the bonding quality of the donor and handle wafers is critical. Any imperfections in surface cleanliness, flatness, or roughness can lead to incomplete bonding, resulting in defects or voids in the final silicon-on-insulator (SOI) structure. This dependence on meticulous control makes the process both expensive and time-consuming, especially for large-scale manufacturing.
Scalability of New Materials
Recent advancements in ultrathin ferroelectric materials show promise; however, challenges in producing these materials at scale remain. Current methods yield only single transistors, hindering mass production capabilities. The transition from lab-scale demonstrations to wafer-scale manufacturing is essential for broader application in electronics, yet presents a significant barrier to scalability.
Integration with Flexible Substrates
The challenge of integrating ultrathin materials with flexible substrates further complicates the landscape. Traditional heat-intensive processes required for device formation are not compatible with flexible materials, which can melt or decompose. While innovative multi-step processes using solid substrates have emerged, they still face hurdles in achieving the desired performance and flexibility for practical applications in the Internet of Things and wearable technologies.
I do not even know how I ended up here but I thought this post was great I dont know who you are but definitely youre going to a famous blogger if you arent already Cheers